Marjory Moreau
Last week, my colleague, Scott Slattery, presented a webinar on in vitro inhalation point-of-contact toxicity testing with vapors. It brought me back to when I started working with Scott last year on this project. As a physiologically based pharmacokinetic modeler, the only time I worked in a lab was during my Ph.D. At that time, I worked with animals, not cells, so I learned a LOT about in vitro inhalation exposure. Scott, our colleagues Asayah Barnwell and Sage Corzine, and I along with my fellow modelers, Mel Andersen, and Jeff Fisher, worked together to develop in vitro and in silico alternatives to replace traditional in vivo methods for inhalation toxicity assessment. I want to share these with you because inhalation dosimetry is still a challenge considering that in inhalation toxicology, the delivered dose depends on the exposure concentration and duration, chemical properties, and portal of entry parameters such as airway architecture, ventilation rate or diffusion across lung tissues. Here I highlight some of the key points of Scott’s webinar, emphasizing how emerging models are more biologically relevant to humans and provide cost and time savings in safety testing.
Airways, Toxins, and Modeling
Chemicals can be inhaled in different physical states, including gases and vapors, that can cause a wide range of adverse health effects, ranging from portal of entry (respiratory tract) irritation to systemic diseases. The airway epithelium is at the interface, where inhaled chemicals interact with lung tissue. In addition to serving as the initial barrier and first line of defense from inhaled chemicals, the airway epithelium serves as a proinflammatory signaling platform to recruit and activate immune cells (e.g., neutrophils and macrophages) following toxic chemical exposure. The airway epithelium is diverse. It is composed of different cell types or different proportions of those cell types in different regions of the airway. Each distinct tissue may have distinct sensitivity to toxins, both because the unique physiological characteristics of each tissue may create differential toxicodynamics, as well as because the different localization of each tissue within the airway may cause differential exposure after inhalation of an airborne toxin. The objective of Scott’s work is to assess whether in vivo points of departure (PODs) for point-of-contact inhalation toxicants can be predicted by an approach that combines in vitro exposures of organotypic cultures to airborne test compounds and in silico modeling of airway dosimetry.
Highly Representative Airway Epithelial Structure
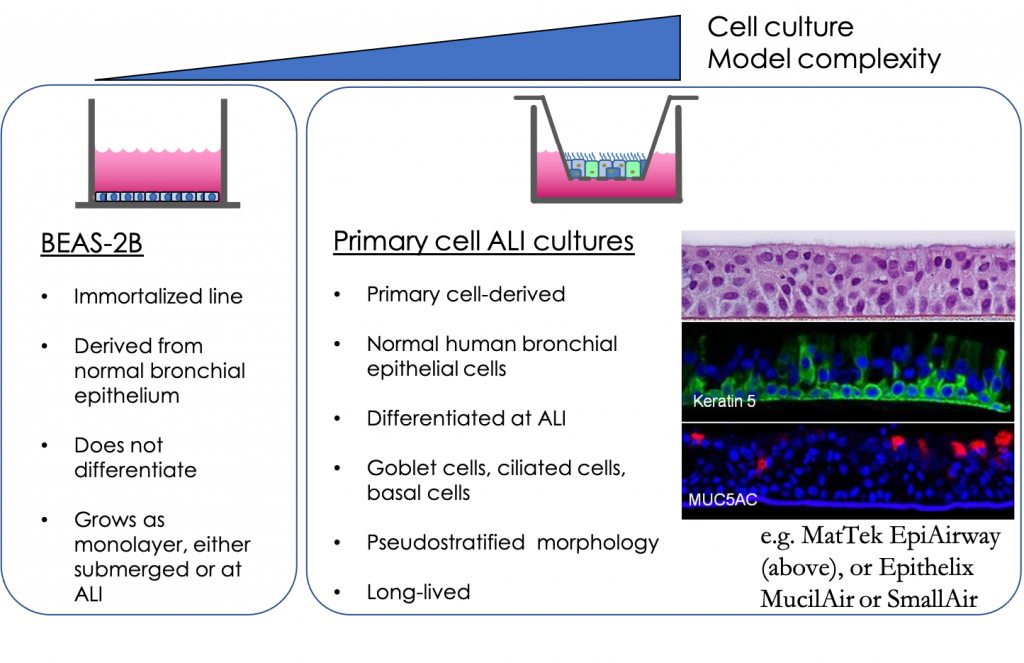
Cell-line based monoculture models are readily accessible, straightforward, and practical; however, these systems lack architectural support and heterogeneity of lung cell type characteristic of the lung in vivo, which makes it difficult to recapitulate physiological and pathophysiological functions in vitro. Most airway epithelial cell lines are not known to undergo differentiation; however, when grown at air–liquid interface (ALI) in the presence of the appropriate medium, primary airway epithelial cells will polarize and form a pseudostratified epithelium composed of basal, ciliated, and goblet cells that closely resemble the human in vivo airway epithelium. Fully differentiated culture exhibits tight junctions, beating cilia, and secrete mucin and protective mediators (e.g., antimicrobial peptides and proinflammatory cytokines). The resulting differentiated model is highly representative of the airway epithelial structure and function in vivo.
Representative Cell Culture Models for a Diverse Airway
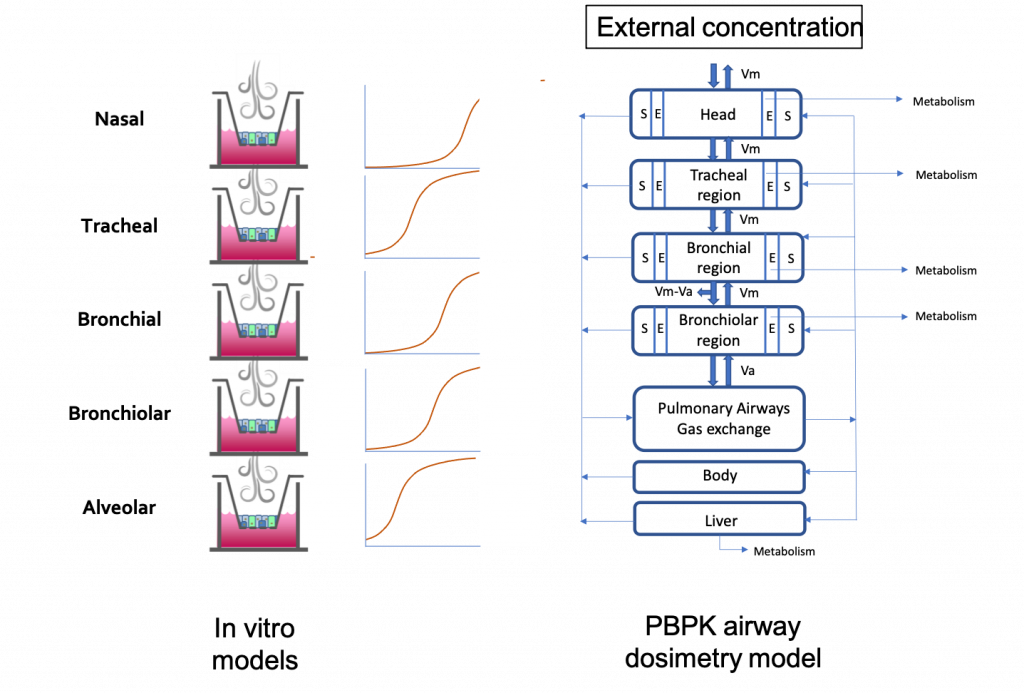
Scott’s team identified five in vitro cell culture models of human airway epithelia – MucilAir of nasal, tracheal, or bronchial origin, SmallAir, and EpiAlveolar – that represent five different regions of the airway epithelium – nasal, tracheal, bronchial, bronchiolar, and alveolar. They exposed these five models to a concentration range of a toxic vapor, 1,3-dichloropropene and monitored toxicity in these cultures 24 hours after exposure using the TEER assay (for epithelial barrier integrity) and the LDH-release assay (for cytotoxicity). In vitro points of departure were identified for each of these tissues.
Bridging In Vitro and PBPK models
The disposition of the gas in the body is determined by different factors like the rate of transfer from the airstream to the lung tissues, absorption into the blood, and the rate of elimination by chemical reaction, exhalation, metabolism, and excretion. This means that the exposure in the most distal epithelium in the alveolar region might be reduced from the upper airways because the vapor is quickly removed by blood flow and, with high metabolism and systemic clearance in the body, would not reach an equilibrium. A physiologically based pharmacokinetic model was developed to predict site-specific lumen air concentrations of 1,3-dichloropropene after inhalation of a given external concentration. To bridge the in vitro systems and the PBPK model, we considered the 1,3-DCP concentration in the exposure air within the in vitro system for each tissue to be equivalent to the 1,3-DCP concentration in the air of the lumen above the same tissue within the PBPK model. For each tissue, reverse dosimetry was performed with the PBPK model to calculate the rat equivalent inhalation concentration that would produce, after a 4-hour exposure, a 1,3-DCP concentration within the lumen adjacent to that tissue that was equivalent to the in vitro POD for the same tissue. The exposure concentrations are in the same order of magnitude in the upper respiratory tract but when we go down the respiratory tract, the PBPK model accounts for the metabolism and systemic clearance of the 1,3-DCP. This explains the higher exposure concentration required in the PBPK model to reach a particular in vitro concentration.
A Better Representation for Risk Assessment
In vitro testing is an efficient way to identify potential toxicity of chemicals. However, in vitro assay concentrations are not necessarily representative of in vivo effect. In vitro to in vivo extrapolation using a PBPK model can translate in vitro concentration-effect curves into in vivo dose-response curves that can be compared to in vivo POD. This kind of work is needed to make risk assessment based on in vitro and in silico methods acceptable and applicable.
To learn more, watch our webinar.